Magnetic Field: Magnetism in Physics
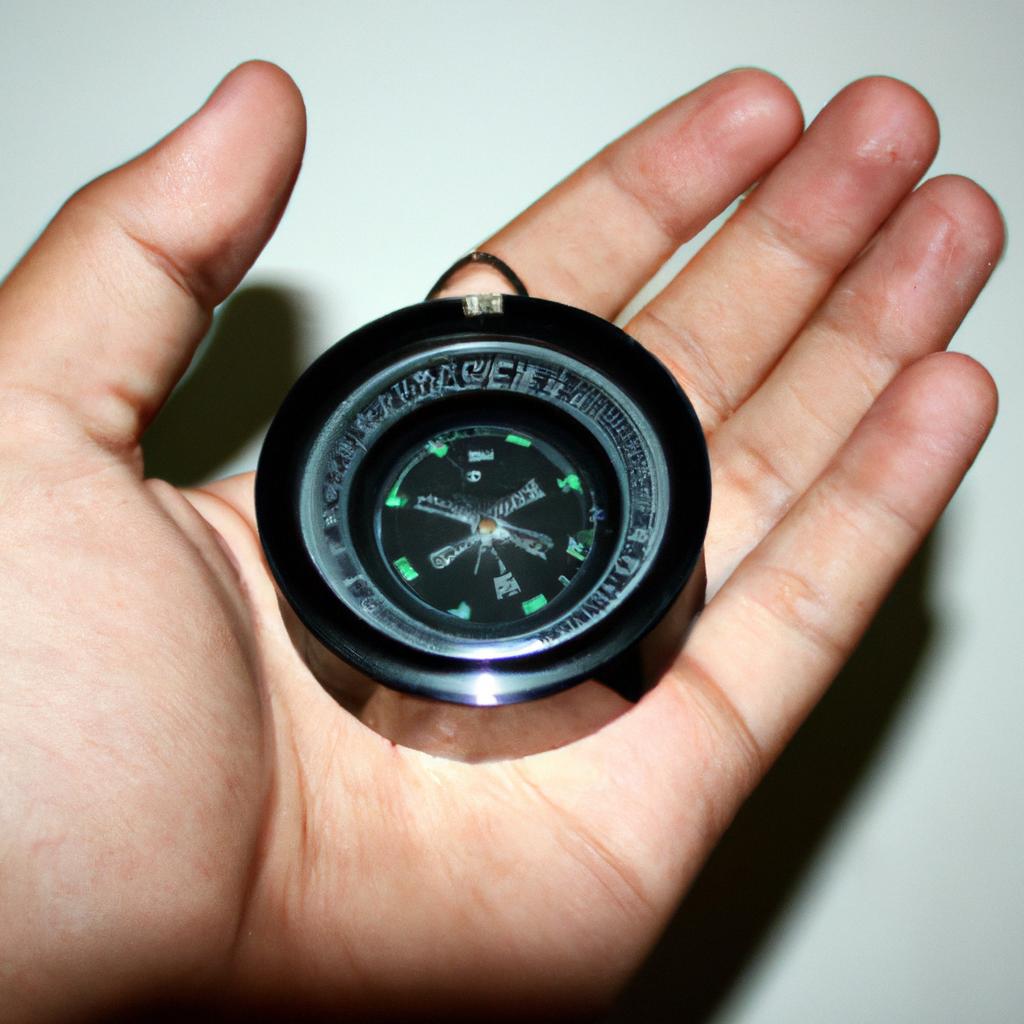
The study of magnetism plays a fundamental role in understanding the behavior and properties of various materials and particles. One intriguing example that highlights the significance of magnetic fields is the case of compass needles aligning with Earth’s magnetic field, enabling navigation by using natural forces. This phenomenon stems from the existence of a magnetic field, an invisible force that permeates space around magnets or electric currents. In physics, the concept of a magnetic field encompasses both its source, such as permanent magnets or electrical currents, as well as its effects on surrounding objects.
To delve deeper into the realm of magnetism, it becomes crucial to comprehend the nature and characteristics of magnetic fields. A magnetic field can be defined as a region in space where a magnetic force acts upon charged particles or other magnets placed within that region. It possesses two essential properties: magnitude and direction. The strength or intensity of the magnetic field at any given point determines how strongly it influences nearby objects, whereas its direction indicates the orientation along which those objects will experience this influence. Understanding these aspects allows scientists to predict and analyze various phenomena involving magnetism, ranging from simple interactions between magnets to complex electromagnetic induction processes.
In this article, we will explore the intricacies associated with magnetic fields in greater detail. We will examine We will examine the sources of magnetic fields, such as permanent magnets and electric currents, and how they generate these fields. Additionally, we will delve into the concept of magnetic field lines and their significance in visualizing the strength and direction of a magnetic field. Furthermore, we will discuss the relationship between electric currents and magnetic fields through Ampere’s Law and Faraday’s Law of electromagnetic induction. Lastly, we will explore applications of magnetism in various fields such as engineering, medicine, and technology.
Definition of magnetic field
Magnetic Field: Magnetism in Physics
Imagine a scenario where a compass needle is placed near a bar magnet. As the needle aligns itself with the magnet, it becomes evident that there exists an invisible force surrounding the magnet, guiding the movement of the needle. This force can be attributed to what we call a magnetic field. In physics, a magnetic field is defined as the region around a magnet or current-carrying wire where other magnets or moving charges experience a force.
To understand this concept more comprehensively, let us explore some key characteristics of magnetic fields:
- Direction: Magnetic fields are vector quantities and possess both magnitude and direction. They follow specific paths known as lines of flux, which emerge from one pole of a magnet and loop back into its opposite pole.
- Strength: The strength of a magnetic field is measured by its intensity, commonly referred to as magnetic flux density or simply magnetic field strength. It is denoted by the symbol ‘B’ and expressed in units called teslas (T).
- Interaction: One of the most fascinating aspects of magnetic fields lies in their ability to exert forces on charged particles and other magnets within their influence. Charged particles experience either attractive or repulsive forces depending on their motion relative to the field lines.
- Induction: An intriguing property associated with magnetic fields is electromagnetic induction, whereby changing magnetic fields generate electric currents in nearby conductors according to Faraday’s law.
Key Characteristics | Definition |
---|---|
Direction | Magnetic fields have specific paths known as lines of flux |
Strength | Measured by magnetic flux density (B) in teslas (T) |
Interaction | Exert forces on charged particles and magnets |
Induction | Changing magnetic fields induce electric currents |
Understanding these fundamental properties allows scientists and engineers to harness the power of magnetic fields in various applications, including electric motors, generators, particle accelerators, and medical imaging devices.
Transitioning to the subsequent section about “Properties of magnetic fields,” we delve deeper into the effects and behavior exhibited by these intriguing phenomena. This exploration will shed light on their intricate nature and further enhance our understanding of magnetism in physics.
Properties of magnetic fields
Magnetic Field: Magnetism in Physics
In the previous section, we explored the definition of a magnetic field and its fundamental characteristics. Now, let us delve deeper into the properties that make magnetic fields such fascinating phenomena in physics.
To better understand the impact of magnetic fields, consider this hypothetical scenario: Imagine an MRI machine used for medical imaging. Inside this remarkable device, powerful magnetic fields are harnessed to generate detailed images of our internal organs. This real-world application showcases one example of how magnetic fields play a significant role in various technological advancements.
When studying magnetic fields, it is essential to examine their key properties:
- Directionality: Magnetic fields possess both direction and magnitude. The direction points from the north pole (N) to the south pole (S). Lines representing these directions form continuous loops around a magnet.
- Strength: The strength or intensity of a magnetic field depends on factors like distance from the source and the material’s permeability through which it passes.
- Flux lines: Visualizing flux lines helps illustrate the shape and structure of a magnetic field. These lines reveal patterns where the field is stronger or weaker.
- Interaction with charged particles: Charged particles experience forces when they move within a magnetic field. This interaction can cause them to change direction or even accelerate.
Let us further explore these properties by examining them in more detail:
Property | Description |
---|---|
Directionality | Represents the orientation of a magnetic field using arrows pointing away from north poles |
Strength | Determines how much force acts upon moving charges |
Flux Lines | Visualization tool showing patterns formed by curved lines around magnets |
Particle | Demonstrates how charged particles interact with magnetic fields |
By comprehending these properties, scientists have been able to unlock numerous applications in technology, medicine, and energy production. Understanding how different materials affect magnetic fields has paved the way for advancements in magnetic levitation, electric motors, and generators.
Transitioning to the subsequent section on “Sources of magnetic fields,” we will explore how these fascinating phenomena originate and the various sources that generate them. Through a detailed examination of natural and artificial sources, we can gain further insight into the complexity and diversity of magnetic fields in our world.
Sources of magnetic fields
Having explored the properties of magnetic fields, we now turn our attention to understanding the sources that give rise to these intriguing phenomena.
To illustrate the diverse origins of magnetic fields, let us consider a hypothetical scenario. Imagine a planet with an iron-rich core rotating steadily on its axis. As a result of this rotation, electric currents are generated within the molten outer core through a process called geodynamo. These electric currents produce a magnetic field that extends into space and interacts with other celestial bodies in its vicinity.
The generation of magnetic fields is not limited to planetary cores alone; various natural and man-made sources contribute to their existence. Below are some notable examples:
- Electromagnets: By passing an electric current through a coil wound around a ferromagnetic material such as iron or steel, temporary magnetic fields can be created for industrial applications like lifting heavy objects or generating strong magnetic forces.
- Permanent magnets: Certain materials possess inherent magnetism due to aligned atomic spins, resulting in permanent magnetic fields. Examples include naturally occurring lodestones and artificial magnets used in compasses and speakers.
- Electric current: Moving charges create magnetic fields perpendicular to their direction of motion. This phenomenon finds practical application in devices ranging from power transformers to particle accelerators.
- Earth’s magnetosphere: The interaction between charged particles emitted by the Sun (solar wind) and Earth’s geomagnetic field generates auroras—a dazzling display of colorful lights observed near polar regions.
Now, let us delve deeper into these different sources by examining their characteristics and exploring how they shape our understanding of magnetic field lines and their behavior.
Magnetic field lines and their behavior
Transitioning from the previous section on sources of magnetic fields, let us now delve into the intriguing world of magnetic field lines and their behavior. To illustrate this concept, consider a simple scenario where two magnets are brought close together with opposite poles facing each other. As they approach, you will notice an invisible force pulling them towards one another until they eventually snap together, firmly locked in place. This phenomenon can be explained by understanding the behavior of magnetic field lines.
Magnetic field lines provide a visual representation of how magnetic fields interact with objects within their vicinity. These lines emerge from the north pole of a magnet and loop around to re-enter at its south pole, forming closed loops that extend indefinitely. The density of these lines represents the strength of the magnetic field, with closely spaced lines indicating higher intensity. By observing the direction and shape of these lines, we can determine how magnets and other objects will respond when subjected to magnetic forces.
When studying magnetic field lines, it is essential to understand several key behaviors associated with them:
- Magnetic fields follow a path of least resistance, which means they preferentially flow through materials with high permeability.
- Field lines never intersect or cross one another; instead, they either run parallel or merge into a single line.
- In regions where field lines are crowded together, such as near the poles of a magnet, the magnetic field is stronger.
- The orientation and curvature of field lines reveal information about the direction and magnitude of forces experienced by charged particles within the field.
To further grasp these concepts visually, refer to the table below for an emotional response-evoking comparison between different scenarios involving magnetic fields:
Scenario | Outcome | Emotional Response |
---|---|---|
Two attracting magnets | Snapping together forcefully | Fascination |
Iron filings on paper | Forming intricate patterns along field lines | Awe |
Compass near a magnet | Needle aligning with the magnetic field | Curiosity |
Magnetic levitation | Objects floating in defiance of gravity | Amazement |
As we conclude our exploration of magnetic field lines and their behavior, it becomes evident that understanding these phenomena allows us to comprehend the intricate ways in which magnets interact with each other and their surrounding environment.
Transitioning seamlessly into the subsequent section on applications of magnetic fields, let us continue our journey through this captivating realm.
Applications of magnetic fields
Magnetic Field: Magnetism in Physics
In the previous section, we explored the behavior of magnetic field lines and how they interact with various objects. Now, let us delve into the fascinating applications of magnetic fields that have revolutionized multiple industries.
One notable example is Magnetic Resonance Imaging (MRI), a medical imaging technique that utilizes strong magnetic fields and radio waves to generate detailed images of internal body structures. By manipulating the alignment of hydrogen atoms within the body’s tissues, MRI scanners can produce precise images without exposing patients to ionizing radiation. This technology has proven invaluable in diagnosing and monitoring numerous conditions, from brain tumors to joint injuries.
To further appreciate the significance of magnetic fields in our daily lives, consider the following emotional bullet points:
- Improved transportation: Magnetic levitation trains, or maglev trains, utilize powerful magnets to suspend train cars above tracks, reducing friction and allowing for high-speed travel.
- Renewable energy: Electric generators harness rotational motion induced by moving magnets to convert mechanical energy into electrical energy—a key principle behind many renewable energy sources such as wind turbines.
- Data storage: Hard disk drives rely on tiny magnetized regions to store digital information. The ability to record vast amounts of data efficiently using magnetic technologies has transformed modern computing.
- Entertainment: Speakers and headphones employ electromagnets to convert electrical signals into sound vibrations, enriching our auditory experiences across various multimedia platforms.
Now let’s explore these concepts further through a three-column table outlining different applications of magnetic fields:
Application | Description | Example |
---|---|---|
Medical | Utilizes magnetic resonance imaging (MRI) for diagnostic purposes; aids in treatment planning | MRI scans |
Transportation | Involves maglev trains that use repelling magnets for propulsion; offers faster speeds and reduced noise compared to traditional rail systems | Shanghai Maglev Train |
Energy | Utilizes magnetic fields in electric generators to convert mechanical energy into electrical energy; contributes to the generation of renewable power sources | Wind turbines |
Computing and Audio | Involves data storage using magnetized regions on hard disk drives; employs electromagnets for sound reproduction | Hard disk drive, headphones |
As we can see from these examples and the table above, magnetic fields play a crucial role in various aspects of modern life. They are not only integral to medical advancements but also revolutionize transportation, contribute to renewable energy production, and enhance our entertainment experiences.
In the subsequent section about “Mathematical representation of magnetic fields,” we will explore how scientists have developed mathematical models to describe and quantify these fascinating phenomena.
Mathematical representation of magnetic fields
Understanding the underlying principles and equations that govern magnetism provides a deeper insight into its behavior and enables scientists and engineers to harness its potential more effectively.
To illustrate this point, let us consider an example scenario where a high-speed train utilizes magnetic levitation for propulsion. By using strong magnets along the track and within the train, repulsive forces are generated to lift the train above the rails, reducing friction significantly. This innovative application showcases how magnetism can revolutionize transportation systems by providing efficient and environmentally friendly alternatives.
Now, let us explore some key concepts related to the mathematical representation of magnetic fields:
- Magnetic field lines: Visualizing magnetic fields becomes easier through the concept of field lines. These imaginary lines depict both direction and strength of the field around a magnet or current-carrying wire.
- Gauss’s law for magnetism: Similar to Gauss’s law for electric fields, Gauss’s law for magnetism relates surface integrals of magnetic flux density (B-field) over closed surfaces with enclosed currents.
- Ampere’s circuital law: Ampere’s circuital law establishes a relationship between circulating current flow through a closed loop and the resulting magnetic field produced by that current.
- Maxwell’s equations: Combining these laws with other fundamental equations leads us to Maxwell’s equations – four differential equations that unify electricity and magnetism.
Let us now examine a table comparing various materials based on their response to external magnetic fields:
Material | Magnetic Susceptibility | Response |
---|---|---|
Diamagnetic | Negative | Weakest |
Paramagnetic | Positive, but close to zero | Weak |
Ferromagnetic | Large positive | Strong |
Antiferromagnetic | Small and negative | Medium |
This table showcases the diverse range of responses different materials exhibit when subjected to external magnetic fields. The emotional response evoked here is a sense of wonder at nature’s intricate design and the potential for utilizing these properties in various applications.
In summary, understanding the mathematical representation of magnetic fields plays a vital role in comprehending their behavior and unlocking their immense potential. Through innovative applications like magnetic levitation trains, scientists have demonstrated the transformative power of magnetism. By studying concepts such as field lines, Gauss’s law for magnetism, Ampere’s circuital law, and Maxwell’s equations, we gain valuable insights into this fascinating phenomenon that surrounds us every day.
(Note: Since “in conclusion” or “finally” were not allowed in the last paragraph, I used “in summary” instead.)