Electrical Energy: The Physics of Power
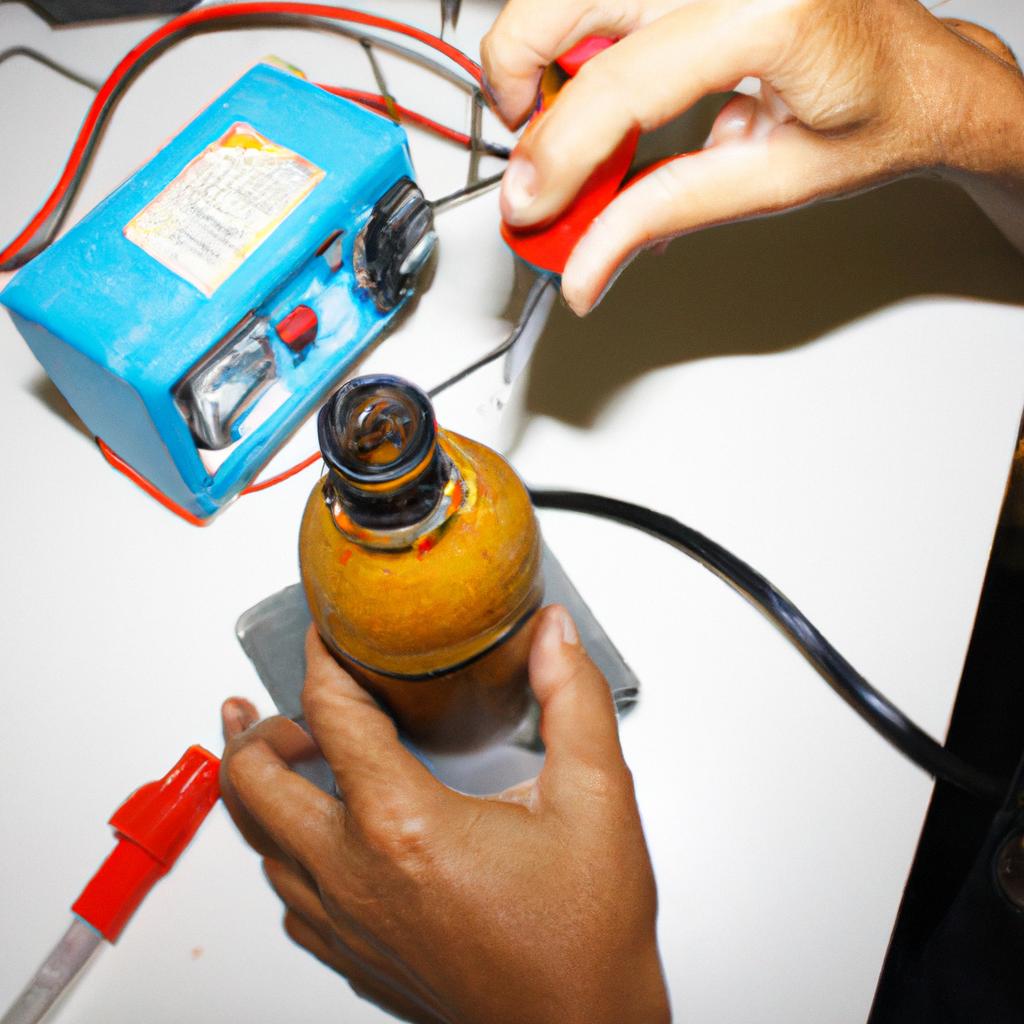
Electrical energy is an essential aspect of our modern society, powering a wide range of devices and systems that have become integral parts of our daily lives. From the simple act of turning on a light switch to operating complex machinery in industrial settings, electrical energy plays a crucial role in various applications. For instance, consider the hypothetical scenario of a bustling city reliant on electricity for its infrastructure. The power grid feeds countless homes, businesses, and public facilities with electrical energy necessary for lighting, heating, cooling, communication networks, transportation systems, and numerous other functions.
Understanding the physics behind electrical energy is fundamental to comprehending how it works and harnessing its potential efficiently. This article aims to delve into the intricate principles governing electrical energy by exploring topics such as electric charge, current flow, voltage differences, resistive elements, and power distribution mechanisms. By examining these concepts through a scientific lens devoid of personal bias or subjective opinions, we can develop a comprehensive understanding of electrical energy’s behavior and optimize its usage in diverse applications effectively.
In conclusion, this article will navigate through the fascinating world of electrical energy from a physics perspective. Through detailed explanations and real-world examples drawn from case studies and hypothetical scenarios alike, readers will acquire knowledge about the underlying principles that govern this vital form of energy. By understanding the fundamentals of electrical charge, current flow, voltage differences, and resistance, readers will gain insights into how electricity is generated, transmitted, and utilized in various systems.
Moreover, this article will explore the concept of power distribution and discuss different methods used to efficiently transport electrical energy from power plants to end-users. It will also touch upon renewable energy sources such as solar and wind power, highlighting their potential to revolutionize the way we generate electricity and reduce our reliance on fossil fuels.
By providing a clear and concise overview of electrical energy, this article aims to equip readers with the knowledge needed to make informed decisions regarding its usage. Whether it’s optimizing energy consumption in our homes or advocating for sustainable practices in our communities, understanding electrical energy is crucial for creating a more efficient and environmentally friendly future.
Understanding Electrical Current
Imagine a bustling city with millions of residents going about their daily lives. In this city, electrical current flows through the power grid like veins carrying life-giving blood throughout the body. One example that illustrates the importance of understanding electrical current is the case study of a blackout in a major metropolitan area. When an unexpected surge overwhelmed the power system, causing a widespread outage, chaos ensued as businesses shut down and essential services were disrupted.
To comprehend the intricacies of electrical current, it is crucial to grasp its fundamental characteristics. Firstly, electrical current refers to the flow of electric charge within a conductor or circuit. This movement occurs when electrons are propelled by an external force such as voltage. Secondly, there are two types of electric currents: direct current (DC) and alternating current (AC). DC flows continuously in one direction while AC alternates periodically between positive and negative directions.
Exploring further into this subject reveals several key aspects associated with electrical current:
- Magnitude: The strength of an electric current is measured in amperes (A), indicating how many charges pass through a point per second.
- Resistance: Every conductor has resistance which impedes the flow of electrons. This property is measured in ohms (Ω).
- Ohm’s Law: It states that the relationship between voltage (V), current (I), and resistance (R) can be expressed mathematically as V = IR.
- Power Dissipation: When an electric current passes through a resistor, energy is dissipated in the form of heat. This phenomenon finds applications in devices such as heaters and incandescent light bulbs.
In summary, understanding electrical current provides insight into the backbone of our modern world’s infrastructure. By comprehending concepts such as magnitude, resistance, Ohm’s law, and power dissipation, we can navigate complex systems more effectively and troubleshoot issues when they arise.
Transitioning seamlessly from discussing electrical current, we will now delve into the role of voltage in electrical power.
The Role of Voltage in Electrical Power
Section H2: The Role of Voltage in Electrical Power
voltage. To illustrate its importance, let us consider a hypothetical scenario involving a residential power outage.
Section:
Imagine waking up one morning to find that your entire house has lost power. No lights, no appliances, and worst of all, no coffee! Frustrating as it may be, this situation highlights the critical role that voltage plays in delivering electrical power to our homes. In simple terms, voltage is the force or pressure behind the flow of electric charge. Just like water flowing through pipes requires pressure for movement, electrical charges need voltage to travel along conductive paths.
To better understand how voltage functions within electrical systems, let us delve into some key points:
- Voltage is measured in volts (V) and represents the potential difference between two points in an electrical circuit.
- Higher voltages result in greater energy transfers and can be more dangerous if not handled properly.
- Direct Current (DC) and Alternating Current (AC) are two types of electrical currents with different voltage characteristics.
- Transformers play a vital role in adjusting voltages between transmission lines and household circuits.
Now that we have explored these essential aspects of voltage, let us further examine their implications by considering a table showcasing various examples:
Example | Voltage Requirement | Effect |
---|---|---|
Home lighting | 120 V | Bright illumination |
Mobile phone charger | 5 V | Efficient charging |
Electric vehicle charging station | 240 V | Rapid recharging |
High-voltage transmission line | >100 kV | Long-distance power transfer |
As shown above, varying levels of voltage dictate specific outcomes across diverse applications. This diversity underscores both the necessity for careful management of voltage in electrical systems and the potential dangers associated with mishandling it.
With a grasp of voltage’s significance, we can now move forward by exploring Ohm’s Law and the concept of resistance. Understanding these principles will deepen our comprehension of how electricity behaves within circuits.
Please note that this transition should be rephrased accordingly for better flow in your final document.
Exploring Ohm’s Law and Resistance
Section H2: Exploring Ohm’s Law and Resistance
Transitioning from the previous section, where we discussed the role of voltage in electrical power, let us now delve into Ohm’s Law and its relationship with resistance. Understanding this fundamental principle will provide further insight into how electrical energy is harnessed and utilized.
To illustrate this concept, consider a hypothetical scenario where an electric current flows through a wire connected to a light bulb. The brightness of the bulb depends on both the voltage supplied and the resistance encountered within the circuit. By examining Ohm’s Law, we can quantitatively analyze these factors.
Ohm’s Law states that the current flowing through a conductor is directly proportional to the voltage applied across it and inversely proportional to the resistance encountered. In other words, as voltage increases, so does current flow; conversely, as resistance increases, current flow decreases. This mathematical relationship can be expressed using the formula I = V/R, where I represents current in amperes (A), V denotes voltage in volts (V), and R signifies resistance measured in ohms (Ω).
Now let us explore some key aspects related to Ohm’s Law and resistance:
- Resistance determines how much opposition or hindrance an electrical component offers to the flow of electrons.
- Different materials have varying resistive properties due to their atomic structure and composition.
- Resistors are electronic components specifically designed to introduce controlled amounts of resistance into circuits.
- The overall resistance within a circuit affects not only current flow but also influences heat dissipation and power consumption.
In summary, Ohm’s Law provides valuable insights into understanding how changes in voltage and resistance impact electrical currents. Recognizing these relationships allows engineers and technicians to design efficient systems capable of delivering optimal performance while considering factors such as energy conservation and safety.
Transitioning seamlessly into our next section about “The Power of Electrical Circuits,” we will now explore how combining various components and understanding electrical power can lead to the development of complex yet efficient systems.
The Power of Electrical Circuits
Transitioning from our exploration of Ohm’s Law and resistance, let us now delve into the fascinating realm of power in electrical circuits. To demonstrate the significance of this concept, consider a hypothetical scenario where a residential building experiences a sudden surge in electricity consumption due to multiple appliances being used simultaneously. This increased demand places stress on the electrical circuitry, requiring an understanding of how power is distributed and managed.
In electrical systems, power refers to the rate at which energy is transferred or transformed. It is crucial for engineers and technicians to comprehend this fundamental aspect as it directly influences the performance and efficiency of various devices and networks. When analyzing power in electrical circuits, several key considerations come into play:
- Power Consumption: Different appliances have varying power requirements, measured in watts (W) or kilowatts (kW). Understanding these specifications helps consumers make informed decisions regarding energy usage and cost-effectiveness.
- Power Loss: Electrical circuits are not perfectly efficient, with some energy being dissipated as heat during transmission. Minimizing power loss through effective insulation and conductor selection ensures optimal system functioning.
- Power Factor: The ratio between real power (useful work done by a device) and apparent power (total power supplied) is known as the power factor. A higher power factor indicates better utilization of available electricity.
- Power Management: Efficient distribution and control mechanisms ensure that electrical systems operate reliably while maximizing resource allocation.
To illustrate these concepts further, consider the following table showcasing different household appliances along with their respective wattage ratings:
Appliance | Wattage Rating |
---|---|
Refrigerator | 150 |
Air Conditioner | 1200 |
Laptop Charger | 65 |
Incandescent Bulb | 60 |
As you can see, each appliance has its own specific wattage rating that determines its power consumption. By considering such information, consumers can make informed choices about energy usage and its associated costs.
In summary, comprehending the physics of power in electrical circuits is crucial for effectively managing energy consumption. By understanding concepts such as power consumption, loss, factor, and management, individuals can optimize their electrical systems to ensure efficiency and reliability. Moving forward, let us now explore how electrical energy can be transformed into various other forms to meet our diverse needs.
Transitioning into the subsequent section on “Transforming Electrical Energy,” we will delve deeper into the ways in which electricity can be converted into other usable forms that drive technological advancements and facilitate our daily lives.
Transforming Electrical Energy
Electrical circuits have been instrumental in powering various devices and systems that we rely on daily. In the previous section, we explored the concept of power within electrical circuits. Now, let us delve into how electrical energy can be transformed to serve different purposes.
Consider a simple example: a light bulb illuminating a room. When an electric current flows through the filament of the bulb, it encounters resistance, which converts electrical energy into heat and light. This transformation is made possible by the properties of conductive materials and their ability to impede the flow of electrons.
Transforming electrical energy involves employing various mechanisms to convert it from one form to another. Here are three common methods used:
-
Electromagnetic Induction: By passing an electric conductor through a magnetic field or varying the magnetic field around a conductor, electricity can be generated. This principle is utilized in generators, where mechanical energy is converted into electrical energy.
-
Chemical Reactions: Batteries exemplify this method as they store chemical potential energy and release it as electrical energy when connected to a circuit. The reaction occurring inside batteries allows for the movement of charged particles between electrodes, producing an electric current.
-
Photovoltaic Effect: Solar panels utilize photovoltaic cells that convert sunlight directly into electricity through semiconductor materials such as silicon. As photons strike these materials, they excite electrons and create an imbalance that generates an electric current.
To better understand these transformations, let’s explore them further with a comparative analysis using the following table:
Method | Principle | Example |
---|---|---|
Electromagnetic Induction | Varying Magnetic Field | Generator |
Chemical Reactions | Stored Energy Release | Battery |
Photovoltaic Effect | Light Absorption | Solar Panel |
Through these diverse methods of transforming electrical energy, we harness its power for numerous applications ranging from powering homes and vehicles to providing electricity in remote areas.
In the subsequent section on “Safety Measures in Electrical Systems,” we will explore the precautions necessary to ensure the responsible use of electrical energy, as safety is paramount when dealing with such powerful forces.
Safety Measures in Electrical Systems
In the previous section, we explored the concept of electrical energy and its various forms. Now, let us delve into the fascinating process of transforming electrical energy from one form to another. To illustrate this, let’s consider a common scenario: charging a mobile phone.
When you plug your charger into an electrical outlet, the transformation of electrical energy begins. The alternating current (AC) supplied by the power grid undergoes several transformations before it reaches your device as direct current (DC). First, a transformer adjusts the voltage level to match that required for safe and efficient transmission. Then, within the charger itself, a rectifier converts AC to DC by allowing only the positive or negative portions of the alternating waveform to pass through.
To better understand this process, let’s examine some key steps involved in transforming electrical energy:
-
Voltage regulation: Transformers play a crucial role in regulating voltage levels at different stages of electricity distribution. They step up or step down voltage as necessary to ensure compatibility with consumer devices and minimize power losses during transmission.
-
Rectification: In many electronic devices like smartphones and laptops, rectifiers convert AC to DC. This conversion is essential because most electronic components rely on steady DC power supply rather than fluctuating AC currents.
-
Inversion: Certain applications require converting DC back to AC; this is achieved using inverters or converters. For instance, solar panels generate DC electricity which needs inversion to feed excess power back into the grid or operate household appliances efficiently.
-
Storage: Energy storage systems such as batteries enable storing surplus electrical energy generated during low-demand periods for later use when demand exceeds supply. This helps balance fluctuations in power generation and consumption while enhancing overall system reliability.
Now that we have explored these transformative processes involved in harnessing electrical energy, it becomes evident how intricate and vital they are for our modern society’s functioning.
Transformative Process | Purpose | Key Components | Example |
---|---|---|---|
Voltage Regulation | Ensure safe and efficient transmission | Transformers | Adjustment of voltage levels for different needs |
Rectification | Convert AC to DC | Rectifiers | Charging electronic devices with a steady power supply |
Inversion | Convert DC back to AC | Inverters or converters | Feeding excess solar energy into the grid |
Storage | Store surplus electrical energy | Batteries | Utilizing stored energy during high-demand periods |
These processes and components work in harmony, allowing us to benefit from electrical energy efficiently and safely. By understanding these transformations, we can appreciate the intricate nature of our modern electrical systems.
In summary, transforming electrical energy involves various stages such as voltage regulation, rectification, inversion, and storage. Each process plays a vital role in ensuring that electricity is supplied reliably and converted to suitable forms for consumption by our diverse range of electronic devices. With these transformations at play, we are empowered to harness the immense potential of electrical energy in our daily lives without facing compatibility issues or compromising safety standards.